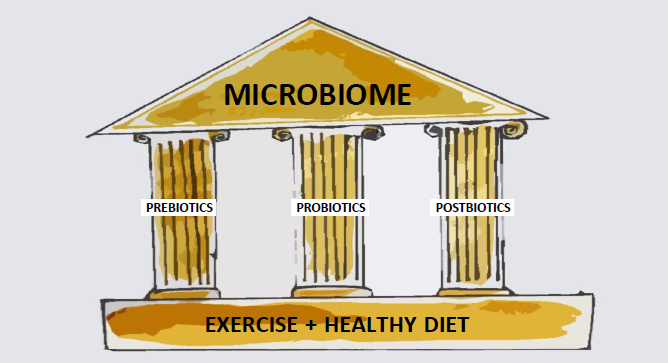
Submission to VIJ 2024-05-26
Keywords
- bacteria; inflammation; microbiome; postbiotic; prebiotic; probiotic
Copyright (c) 2024 Jakub Z˙ ółkiewicz, Aleksandra Marzec, Wong Jest Phia

This work is licensed under a Creative Commons Attribution 4.0 International License.
Abstract
As an imbalance in the intestinal microbiota can lead to the development of several diseases (e.g., type 1 diabetes, cancer, among others), the use of prebiotics, probiotics, and postbiotics to alter the gut microbiome has attracted recent interest. Postbiotics include any substance released by or produced through the metabolic activity of the microorganism, which exerts a beneficial effect on the host, directly or indirectly. As postbiotics do not contain live microorganisms, the risks associated with their intake are minimized. Here, we provided a critical review of postbiotics described in the literature, including their mechanisms of action, clinical characteristics, and potential therapeutic applications. We detailed the pleiotropic effects of postbiotics, including their immunomodulatory, anti-inflammatory, antioxidant, and anti-cancer properties. Although the use of postbiotics is an attractive strategy for altering the microbiome, further study into its efficacy and safety is warranted.
References
- Rodriguez, J.M.; Murphy, K.; Stanton, C.; Ross, R.P.; Kober, O.I.; Juge, N.; Avershina, E.; Rudi, K.; Narbad, A.; Jenmalm, M.C.; et al. The composition of the gut microbiota throughout life, with an emphasis on early life. Microb. Ecol. Health Dis. 2015, 26, 26050. [CrossRef]
- Edwards, S.M.; Cunningham, S.A.; Dunlop, A.L.; Corwin, E.J. The Maternal Gut Microbiome during Pregnancy. MCN Am. J. Matern. Child Nurs. 2017, 42, 310–317. [CrossRef]
- Bastiaanssen, T.F.S.; Cowan, C.S.M.; Claesson, M.J.; Dinan, T.G.; Cryan, J.F. Making Sense of the Microbiome in Psychiatry. Int. J. Neuropsychopharmacol. 2019, 22, 37–52. [CrossRef]
- Derwa, Y.; Gracie, D.J.; Hamlin, P.J.; Ford, A.C. Systematic review with meta-analysis: The efficacy of
- probiotics in inflammatory bowel disease. Aliment. Pharmacol. Ther. 2017, 46, 389–400. [CrossRef]
- Szajewska, H.; Kolodziej, M.; Gieruszczak-Bialek, D.; Skorka, A.; Ruszczynski, M.; Shamir, R. Systematic review with meta-analysis: Lactobacillus rhamnosus GG for treating acute gastroenteritis in children-a 2019 update. Aliment. Pharmacol. Ther. 2019, 49, 1376–1384. [CrossRef]
- Kothari, D.; Patel, S.; Kim, S.-K. Probiotic supplements might not be universally-effective and safe: A review. Biomed. Pharmacother. 2019, 111, 537–547. [CrossRef]
- Tsilingiri, K.; Rescigno, M. Postbiotics: What else? Benef. Microbes 2013, 4, 101–107. [CrossRef]
- Klemashevich, C.; Wu, C.; Howsmon, D.; Alaniz, R.C.; Lee, K.; Jayaraman, A. Rational identification of diet-derived postbiotics for improving intestinal microbiota function. Curr. Opin. Biotechnol. 2014, 26, 85–90. [CrossRef] [PubMed]
- De Marco, S.; Sichetti, M.; Muradyan, D.; Piccioni, M.; Traina, G.; Pagiotti, R.; Pietrella, D. Probiotic Cell-Free Supernatants Exhibited Anti-Inflammatory and Antioxidant Activity on Human Gut Epithelial Cells and Macrophages Stimulated with LPS. Evid. Based Complementary Altern. Med. 2018, 2018, 1756308. [CrossRef]
- Escamilla, J.; Lane, M.A.; Maitin, V. Cell-free supernatants from probiotic Lactobacillus casei and Lactobacillus rhamnosus GG decrease colon cancer cell invasion in vitro. Nutr. Cancer 2012, 64, 871–878. [CrossRef]
- Amaretti, A.; di Nunzio, M.; Pompei, A.; Raimondi, S.; Rossi, M.; Bordoni, A. Antioxidant properties of potentially probiotic bacteria: In vitro and in vivo activities. Appl. Microbiol. Biotechnol. 2013, 97, 809–817. [CrossRef]
- Khodaii, Z.; Ghaderian, S.M.H.; Natanzi, M.M. Probiotic Bacteria and their Supernatants Protect Enterocyte Cell Lines from Enteroinvasive Escherichia coli (EIEC) Invasion. Int. J. Mol. Cell. Med. 2017, 6, 183–189. [CrossRef]
- Izuddin, W.I.; Loh, T.C.; Foo, H.L.; Samsudin, A.A.; Humam, A.M. Postbiotic L. plantarum RG14 improves ruminal epithelium growth, immune status and upregulates the intestinal barrier function in post-weaning lambs. Sci. Rep. 2019, 9, 9938. [CrossRef]
- West, C.; Stanisz, A.M.; Wong, A.; Kunze, W.A. Effects of Saccharomyces cerevisiae or boulardii yeasts on acute stress induced intestinal dysmotility. World J. Gastroenterol. 2016, 22, 10532–10544. [CrossRef]
- Canonici, A.; Siret, C.; Pellegrino, E.; Pontier-Bres, R.; Pouyet, L.; Montero, M.P.; Colin, C.; Czerucka, D.; Rigot, V.; Andre, F. Saccharomyces boulardii improves intestinal cell restitution through activation of the alpha2beta1 integrin collagen receptor. PLoS ONE 2011, 6, e18427. [CrossRef]
- Singh, P.; Saini, P. Food and Health Potentials of Exopolysaccharides Derived from Lactobacilli. Microbiol. Res. J. Int. 2017, 1–14. [CrossRef]
- Makino, S.; Sato, A.; Goto, A.; Nakamura, M.; Ogawa, M.; Chiba, Y.; Hemmi, J.; Kano, H.; Takeda, K.; Okumura, K.; et al. Enhanced natural killer cell activation by exopolysaccharides derived from yogurt fermented with Lactobacillus delbrueckii ssp. bulgaricus OLL1073R-1. J. Dairy Sci. 2016, 99, 915–923. [CrossRef]
- Wang, J.; Wu, T.; Fang, X.; Min, W.; Yang, Z. Characterization and immunomodulatory activity of an exopolysaccharide produced by Lactobacillus plantarum JLK0142 isolated from fermented dairy tofu. Int. J. Biol. Macromol. 2018, 115, 985–993. [CrossRef]
- Lei, X.; Zhang, H.; Hu, Z.; Liang, Y.; Guo, S.; Yang, M.; Du, R.; Wang, X. Immunostimulatory activity of exopolysaccharides from probiotic Lactobacillus casei WXD030 strain as a novel adjuvant in vitro and in vivo. Food Agric. Immunol. 2018, 29, 1086–1105. [CrossRef]
- Khalil, E.S.; Abd Manap, M.Y.; Mustafa, S.; Alhelli, A.M.; Shokryazdan, P. Probiotic Properties of Exopolysaccharide-Producing Lactobacillus Strains Isolated from Tempoyak. Molecules 2018, 23, 398. [CrossRef]
- Li, W.; Ji, J.; Chen, X.; Jiang, M.; Rui, X.; Dong, M. Structural elucidation and antioxidant activities of exopolysaccharides from Lactobacillus helveticus MB2-1. Carbohydr. Polym. 2014, 102, 351–359. [CrossRef] [PubMed]
- Uchida, M.; Ishii, I.; Inoue, C.; Akisato, Y.; Watanabe, K.; Hosoyama, S.; Toida, T.; Ariyoshi, N.; Kitada, M. Kefiran reduces atherosclerosis in rabbits fed a high cholesterol diet. J. Atheroscler. Thromb. 2010, 17, 980–988. [CrossRef] [PubMed]
- Maeda, H.; Zhu, X.; Omura, K.; Suzuki, S.; Kitamura, S. Effects of an exopolysaccharide (kefiran) on lipids, blood pressure, blood glucose, and constipation. Biofactors 2004, 22, 197–200. [CrossRef] [PubMed]
- Brown, G.D.; Taylor, P.R.; Reid, D.M.; Willment, J.A.; Williams, D.L.; Martinez-Pomares, L.; Wong, S.Y.; Gordon, S. Dectin-1 is a major beta-glucan receptor on macrophages. J. Exp. Med. 2002, 196, 407–412. [CrossRef]
- Li, B.; Cai, Y.; Qi, C.; Hansen, R.; Ding, C.; Mitchell, T.C.; Yan, J. Orally administered particulate beta-glucan modulates tumor-capturing dendritic cells and improves antitumor T-cell responses in cancer. Clin. Cancer Res. 2010, 16, 5153–5164. [CrossRef]
- Vetvicka, V.; Vetvickova, J. Glucan supplementation enhances the immune response against an influenza challenge in mice. Ann. Transl. Med. 2015, 3, 22. [CrossRef]
- Garai-Ibabe, G.; Dueñas, M.; Irastorza, A.; Sierra-Filardi, E.; Werning, M.; López, P.; Corbi, A.; Palencia, P. Naturally occurring 2-substituted (1,3)-β-D-glucan producing Lactobacillus suebicus and Pediococcus parvulus strains with potential utility in the production of functional foods. Bioresour. Technol. 2010, 101, 9254–9263. [CrossRef]
- Morifuji, M.; Ichikawa, S.; Kitade, M.; Fukasawa, T.; Asami, Y.; Manabe, Y.; Sugawara, T. Exopolysaccharides from milk fermented by lactic acid bacteria enhance dietary carotenoid bioavailability in humans in a randomized crossover trial and in rats. Am. J. Clin. Nutr. 2020, 111. [CrossRef]
- Jesenak, M.; Urbancek, S.; Majtan, J.; Banovcin, P.; Hercogova, J. β-Glucan-based cream (containing pleuran isolated from pleurotus ostreatus) in supportive treatment of mild-to-moderate atopic dermatitis. J. Dermatol. Treat. 2016, 27, 351–354. [CrossRef]
- Kullisaar, T.; Zilmer, M.; Mikelsaar, M.; Vihalemm, T.; Annuk, H.; Kairane, C.; Kilk, A. Two antioxidative lactobacilli strains as promising probiotics. Int. J. Food Microbiol. 2002, 72, 215–224. [CrossRef]
- Kim, H.; Chae, H.S.; Jeong, S.G.; Ham, J.; Im, S.K.; Ahn, C.N.; Lee, J. In vitro Antioxidative Properties of Lactobacilli. Asian Australas. J. Anim. Sci. 2006, 19. [CrossRef]
- Izuddin, W.I.; Humam, A.M.; Loh, T.C.; Foo, H.L.; Samsudin, A.A. Dietary Postbiotic Lactobacillus plantarum Improves Serum and Ruminal Antioxidant Activity and Upregulates Hepatic Antioxidant Enzymes and Ruminal Barrier Function in Post-Weaning Lambs. Antioxidants 2020, 9, 250. [CrossRef] [PubMed]
- LeBlanc, J.G.; del Carmen, S.; Miyoshi, A.; Azevedo, V.; Sesma, F.; Langella, P.; Bermúdez-Humarán, L.G.; Watterlot, L.; Perdigon, G.; de Moreno de LeBlanc, A. Use of superoxide dismutase and catalase producing lactic acid bacteria in TNBS induced Crohn’s disease in mice. J. Biotechnol. 2011, 151, 287–293. [CrossRef] [PubMed]
- Tomusiak-Plebanek, A.; Heczko, P.; Skowron, B.; Baranowska, A.; Okon, K.; Thor, P.J.; Strus, M. Lactobacilli with superoxide dismutase-like or catalase activity are more effective in alleviating inflammation in an inflammatory bowel disease mouse model. Drug Des. Dev. Ther. 2018, 12, 3221–3233. [CrossRef]
- de Moreno de LeBlanc, A.; LeBlanc, J.G.; Perdigon, G.; Miyoshi, A.; Langella, P.; Azevedo, V.; Sesma, F.Oral administration of a catalase-producing Lactococcus lactis can prevent a chemically induced colon cancer in mice. J. Med. Microbiol. 2008, 57, 100–105. [CrossRef]
- van Langevelde, P.; van Dissel, J.T.; Ravensbergen, E.; Appelmelk, B.J.; Schrijver, I.A.; Groeneveld, P.H.Antibiotic-induced release of lipoteichoic acid and peptidoglycan from Staphylococcus aureus: Quantitative measurements and biological reactivities. Antimicrob. Agents Chemother. 1998, 42, 3073–3078. [CrossRef]
- Kim, H.G.; Kim, N.R.; Gim, M.G.; Lee, J.M.; Lee, S.Y.; Ko, M.Y.; Kim, J.Y.; Han, S.H.; Chung, D.K. Lipoteichoic acid isolated from Lactobacillus plantarum inhibits lipopolysaccharide-induced TNF-alpha production in THP-1 cells and endotoxin shock in mice. J. Immunol. 2008, 180, 2553–2561. [CrossRef]
- Kaji, R.; Kiyoshima-Shibata, J.; Nagaoka, M.; Nanno, M.; Shida, K. Bacterial Teichoic Acids Reverse Predominant IL-12 Production Induced by Certain Lactobacillus Strains into Predominant IL-10 Production via TLR2-Dependent ERK Activation in Macrophages. J. Immunol. (Baltim. Md. 1950) 2010, 184, 3505–3513. [CrossRef]
- Zadeh, M.; Khan, M.; Goh, Y.; Selle, K.; Owen, J.; Klaenhammer, T.; Mohamadzadeh, M. Induction of intestinal pro-inflammatory immune responses by lipoteichoic acid. J. Inflamm. (Lond. Engl.) 2012, 9, 7. [CrossRef] [PubMed]
- Schauber, J.; Gallo, R. Antimicrobial peptides and skin immune defense system. J. Allergy Clin. Immunol.2009, 124, R13–R18. [CrossRef] [PubMed]
- Wang, Z.; MacLeod, D.T.; Di Nardo, A. Commensal bacteria lipoteichoic acid increases skin mast cell antimicrobial activity against vaccinia viruses. J. Immunol. 2012, 189, 1551–1558. [CrossRef] [PubMed]
- Okamoto, M.; Ohe, G.; Oshikawa, T.; Furuichi, S.; Nishikawa, H.; Tano, T.; Ahmed, S.; Yoshida, H.; Moriya, Y.; Saito, M.; et al. Enhancement of anti-cancer immunity by a lipoteichoic-acid-related molecule isolated from a penicillin-killed group A Streptococcus. Cancer Immunol. Immunother. 2001, 50, 408–416. [CrossRef] [PubMed]
- Lebeer, S.; Claes, I.J.J.; Vanderleyden, J. Anti-inflammatory potential of probiotics: Lipoteichoic acid makes a difference. Trends Microbiol. 2012, 20, 5–10. [CrossRef] [PubMed]
- Lee, C.; Kim, B.G.; Kim, J.H.; Chun, J.; Im, J.P.; Kim, J.S. Sodium butyrate inhibits the NF-kappa B signaling pathway and histone deacetylation, and attenuates experimental colitis in an IL-10 independent manner. Int. Immunopharmacol. 2017, 51, 47–56. [CrossRef]
- Lührs, H.; Gerke, T.; Müller, J.G.; Melcher, R.; Schauber, J.; Boxberge, F.; Scheppach, W.; Menzel, T. Butyrate inhibits NF-kappa B activation in lamina propria macrophages of patients with ulcerative colitis. Scand. J. Gastroenterol. 2002, 37, 458–466. [CrossRef]
- Kasahara, K.; Krautkramer, K.A.; Org, E.; Romano, K.A.; Kerby, R.L.; Vivas, E.I.; Mehrabian, M.; Denu, J.M.; Bäckhed, F.; Lusis, A.J.; et al. Interactions between Roseburia intestinalis and diet modulate atherogenesis in a murine model. Nat. Microbiol. 2018, 3, 1461–1471. [CrossRef]
- Aoki, R.; Kamikado, K.; Suda, W.; Takii, H.; Mikami, Y.; Suganuma, N.; Hattori, M.; Koga, Y. A proliferative probiotic Bifidobacterium strain in the gut ameliorates progression of metabolic disorders via microbiota modulation and acetate elevation. Sci. Rep. 2017, 7, 43522. [CrossRef]
- Frost, G.; Sleeth, M.L.; Sahuri-Arisoylu, M.; Lizarbe, B.; Cerdan, S.; Brody, L.; Anastasovska, J.; Ghourab, S.; Hankir, M.; Zhang, S.; et al. The short-chain fatty acid acetate reduces appetite via a central homeostatic mechanism. Nat. Commun. 2014, 5, 3611. [CrossRef]
- Fukuda, S.; Toh, H.; Taylor, T.; Ohno, H.; Hattori, M. Acetate-producing bifidobacteria protect the hose for enteropathogenic infection via carbohydrate transporters. Gut Microbes 2012, 3, 449–454. [CrossRef]
- Bush, R.S.; Milligan, L.P. Study of the mechanism of inhibition of ketogenesis by propionate in bovine liver. Can. J. Anim. Sci. 1971, 51, 121–127. [CrossRef]
- Tedelind, S.; Westberg, F.; Kjerrulf, M.; Vidal, A. Anti-inflammatory properties of the short-chain fatty acids acetate and propionate: A study with relevance to inflammatory bowel disease. World J. Gastroenterol. WJG 2007, 13, 2826–2832. [CrossRef] [PubMed]
- Melbye, P.; Olsson, A.; Hansen, T.H.; Søndergaard, H.B.; Bang Oturai, A. Short-chain fatty acids and gut microbiota in multiple sclerosis. Acta Neurol. Scand. 2019, 139, 208–219. [CrossRef]
- Feleszko, W.; Jaworska, J.; Rha, R.D.; Steinhausen, S.; Avagyan, A.; Jaudszus, A.; Ahrens, B.; Groneberg, D.A.; Wahn, U.; Hamelmann, E. Probiotic-induced suppression of allergic sensitization and airway inflammation is associated with an increase of T regulatory-dependent mechanisms in a murine model of asthma. Clin. Exp. Allergy 2007, 37, 498–505. [CrossRef] [PubMed]
- Kearney, S.C.; Dziekiewicz, M.; Feleszko, W. Immunoregulatory and immunostimulatory responses of bacterial lysates in respiratory infections and asthma. Ann. Allergy Asthma Immunol. 2015, 114, 364–369. [CrossRef] [PubMed]
- Schaad, U.B.; Mütterlein, R.; Goffin, H. Immunostimulation with OM-85 in Children with Recurrent Infections of the Upper Respiratory Tract: A Double-Blind, Placebo-Controlled Multicenter Study. Chest 2002, 122, 2042–2049. [CrossRef]
- Yin, J.; Xu, B.; Zeng, X.; Shen, K. Broncho-Vaxom in pediatric recurrent respiratory tract infections: A systematic review and meta-analysis. Int. Immunopharmacol. 2018, 54, 198–209. [CrossRef]
- de Boer, G.; Z˙ ółkiewicz, J.; Strzelec, K.; Ruszczyn´ ski, M.; Hendriks, R.; Braunstahl, G.; Feleszko, W.; Tramper-Stranders, G. Bacterial lysate add-on therapy for the prevention of wheezing episodes and asthma exacerbations: A systematic review and meta-analysis. Eur. Respir. Rev. 2020, in press.
- Emeryk, A.; Bartkowiak-Emeryk, M.; Raus, Z.; Braido, F.; Ferlazzo, G.; Melioli, G. Mechanical bacterial lysate administration prevents exacerbation in allergic asthmatic children—The EOLIA study. Pediatric Allergy Immunol. 2018, 29, 394–401. [CrossRef]
- Cazzola, M.; Capuano, A.; Rogliani, P.; Matera, M.G. Bacterial lysates as a potentially effective approach in preventing acute exacerbation of COPD. Curr. Opin. Pharmacol. 2012, 12, 300–308. [CrossRef]
- Koatz, A.M.; Coe, N.A.; Cicerán, A.; Alter, A.J. Clinical and Immunological Benefits of OM-85 Bacterial Lysate in Patients with Allergic Rhinitis, Asthma, and COPD and Recurrent Respiratory Infections. Lung 2016, 194, 687–697. [CrossRef]
- Bodemer, C.; Guillet, G.; Cambazard, F.; Boralevi, F.; Ballarini, S.; Milliet, C.; Bertuccio, P.; Vecchia, C.; Bach, J.-F.; Prost, Y. Adjuvant treatment with the bacterial lysate (OM-85) improves management of atopic dermatitis: A randomized study. PLoS ONE 2017, 12, e0161555. [CrossRef]
- Morita, Y.; Jounai, K.; Miyake, M.; Inaba, M.; Kanauchi, O. Effect of Heat-Killed Lactobacillus paracasei KW3110 Ingestion on Ocular Disorders Caused by Visual Display Terminal (VDT) Loads: A Randomized, Double-Blind, Placebo-Controlled Parallel-Group Study. Nutrients 2018, 10, 1058. [CrossRef]
- Hsu, C.Y.; Chiu, S.W.; Hong, K.S.; Saver, J.L.; Wu, Y.L.; Lee, J.D.; Lee, M.; Ovbiagele, B. Folic Acid in Stroke Prevention in Countries without Mandatory Folic Acid Food Fortification: A Meta-Analysis of Randomized Controlled Trials. J. Stroke 2018, 20, 99–109. [CrossRef]
- Kok, D.E.; Steegenga, W.T.; Smid, E.J.; Zoetendal, E.G.; Ulrich, C.M.; Kampman, E. Bacterial folate biosynthesis and colorectal cancer risk: More than just a gut feeling. Crit. Rev. Food Sci. Nutr. 2020, 60, 244–256. [CrossRef]
- Ahire, J.J.; Mokashe, N.U.; Patil, H.J.; Chaudhari, B.L. Antioxidative potential of folate producing probiotic Lactobacillus helveticus CD6. J. Food Sci. Technol. 2013, 50, 26–34. [CrossRef]
- Vogel, R.F.; Pavlovic, M.; Ehrmann, M.A.; Wiezer, A.; Liesegang, H.; Offschanka, S.; Voget, S.; Angelov, A.; Bocker, G.; Liebl, W. Genomic analysis reveals Lactobacillus sanfranciscensis as stable element in traditional sourdoughs. Microb. Cell Fact. 2011, 10 (Suppl. 1), S6. [CrossRef]
- Mohammad, M.A.; Molloy, A.; Scott, J.; Hussein, L. Plasma cobalamin and folate and their metabolic markers methylmalonic acid and total homocysteine among Egyptian children before and after nutritional supplementation with the probiotic bacteria Lactobacillus acidophilus in yoghurt matrix. Int. J. Food Sci. Nutr.2006, 57, 470–480. [CrossRef]
- Karl, J.P.; Meydani, M.; Barnett, J.B.; Vanegas, S.M.; Barger, K.; Fu, X.; Goldin, B.; Kane, A.; Rasmussen, H.; Vangay, P.; et al. Fecal concentrations of bacterially derived vitamin K forms are associated with gut microbiota composition but not plasma or fecal cytokine concentrations in healthy adults. Am. J. Clin. Nutr.2017, 106, 1052–1061. [CrossRef]
- Liu, Y.; Hou, Y.; Wang, G.; Zheng, X.; Hao, H. Gut Microbial Metabolites of Aromatic Amino Acids as Signals in Host-Microbe Interplay. Trends Endocrinol. Metab. 2020. [CrossRef]
- Devlin, A.S.; Marcobal, A.; Dodd, D.; Nayfach, S.; Plummer, N.; Meyer, T.; Pollard, K.S.; Sonnenburg, J.L.; Fischbach, M.A. Modulation of a Circulating Uremic Solute via Rational Genetic Manipulation of the Gut Microbiota. Cell Host Microbe 2016, 20, 709–715. [CrossRef]
- Cortes-Martin, A.; Selma, M.V.; Tomas-Barberan, F.A.; Gonzalez-Sarrias, A.; Espin, J.C. Where to Look into the Puzzle of Polyphenols and Health? The Postbiotics and Gut Microbiota Associated with Human Metabotypes. Mol. Nutr. Food Res. 2020, 64, e1900952. [CrossRef]
- Espin, J.C.; Gonzalez-Sarrias, A.; Tomas-Barberan, F.A. The gut microbiota: A key factor in the therapeutic effects of (poly)phenols. Biochem. Pharmacol. 2017, 139, 82–93. [CrossRef]
- Xia, B.; Shi, X.C.; Xie, B.C.; Zhu, M.Q.; Chen, Y.; Chu, X.Y.; Cai, G.H.; Liu, M.; Yang, S.Z.; Mitchell, G.A.; et al. Urolithin A exerts antiobesity effects through enhancing adipose tissue thermogenesis in mice. PLoS Biol.2020, 18, e3000688. [CrossRef]
- Andreux, P.A.; Blanco-Bose, W.; Ryu, D.; Burdet, F.; Ibberson, M.; Aebischer, P.; Auwerx, J.; Singh, A.; Rinsch, C. The mitophagy activator urolithin A is safe and induces a molecular signature of improved mitochondrial and cellular health in humans. Nat. Metab. 2019, 1, 595–603. [CrossRef]
- Yoshikata, R.; Myint, K.Z.Y.; Ohta, H. Effects of Equol Supplement on Bone and Cardiovascular Parameters in Middle-Aged Japanese Women: A Prospective Observational Study. J. Altern. Complement. Med. 2018, 24, 701–708. [CrossRef]
- Tousen, Y.; Ezaki, J.; Fujii, Y.; Ueno, T.; Nishimuta, M.; Ishimi, Y. Natural S-equol decreases bone resorption in postmenopausal, non-equol-producing Japanese women: A pilot randomized, placebo-controlled trial. Menopause 2011, 18, 563–574. [CrossRef]
- Chung, H.; Pamp, S.J.; Hill, J.A.; Surana, N.K.; Edelman, S.M.; Troy, E.B.; Reading, N.C.; Villablanca, E.J.; Wang, S.; Mora, J.R.; et al. Gut Immune Maturation Depends on Colonization with a Host-Specific Microbiota. Cell 2012, 149, 1578–1593. [CrossRef]
- Furusawa, Y.; Obata, Y.; Fukuda, S.; Endo, T.A.; Nakato, G.; Takahashi, D.; Nakanishi, Y.; Uetake, C.; Kato, K.; Kato, T.; et al. Commensal microbe-derived butyrate induces the differentiation of colonic regulatory T cells. Nature 2013, 504, 446–450. [CrossRef]
- Arpaia, N.; Campbell, C.; Fan, X.; Dikiy, S.; van der Veeken, J.; deRoos, P.; Liu, H.; Cross, J.R.; Pfeffer, K.; Coffer, P.J.; et al. Metabolites produced by commensal bacteria promote peripheral regulatory T-cell generation. Nature 2013, 504, 451–455. [CrossRef]
- Jensen, G.; Benson, K.; Carter, S.; Endres, J. GanedenBC30™ cell wall and metabolites: Anti-inflammatory and immune modulating effects in vitro. BMC Immunol. 2010, 11, 15. [CrossRef]
- Hoarau, C.; Martin, L.; Faugaret, D.; Baron, C.; Dauba, A.; Aubert-Jacquin, C.; Velge-Roussel, F.; Lebranchu, Y. Supernatant from Bifidobacterium Differentially Modulates Transduction Signaling Pathways for Biological Functions of Human Dendritic Cells. PLoS ONE 2008, 3, e2753. [CrossRef]
- Ménard, S.; Laharie, D.; Asensio, C.; Vidal-Martinez, T.; Candalh, C.; Rullier, A.; Zerbib, F.; Megraud, F.; Matysiak-Budnik, T.; Heyman, M. Bifidobacterium breve and Streptococcus thermophilus Secretion Products Enhance T Helper 1 Immune Response and Intestinal Barrier in Mice. Exp. Biol. Med. (Maywood, N.J.) 2005, 230, 749–756. [CrossRef]
- Cousin, F.; Jouan-Lanhouet, S.; Dimanche-Boitrel, M.-T.; Corcos, L.; Jan, G. Milk Fermented by Propionibacterium freudenreichii Induces Apoptosis of HGT-1 Human Gastric Cancer Cells. PLoS ONE
- , 7, e31892. [CrossRef]
- Rigo-Adrover, M.D.; Knipping, K.; Garssen, J.; van Limpt, K.; Knol, J.; Franch, À.; Castell, M.; Rodríguez- lagunas, M.J.; Pérez-Cano, F.J. Prevention of Rotavirus Diarrhea in Suckling Rats by a Specific Fermented Milk Concentrate with Prebiotic Mixture. Nutrients 2019, 11, 189. [CrossRef]
- Nocerino, R.; Paparo, L.; Terrin, G.; Pezzella, V.; Amoroso, A.; Cosenza, L.; Cecere, G.; De Marco, G.; Micillo, M.; Albano, F.; et al. Cow’s milk and rice fermented with Lactobacillus paracasei CBA L74 prevent infectious diseases in children: A randomized controlled trial. Clin. Nutr. 2017, 36, 118–125. [CrossRef]
- Malagón-Rojas, J.N.; Mantziari, A.; Salminen, S.; Szajewska, H. Postbiotics for Preventing and Treating Common Infectious Diseases in Children: A Systematic Review. Nutrients 2020, 12, 389. [CrossRef]
- Corsello, G.; Carta, M.; Marinello, R.; Picca, M.; De Marco, G.; Micillo, M.; Ferrara, D.; Vigneri, P.; Cecere, G.; Ferri, P.; et al. Preventive Effect of Cow’s Milk Fermented with Lactobacillus paracasei CBA L74 on Common Infectious Diseases in Children: A Multicenter Randomized Controlled Trial. Nutrients 2017, 9, 669. [CrossRef]
- Mariadason, J.M.; Catto-Smith, A.; Gibson, P.R. Modulation of distal colonic epithelial barrier function by dietary fibre in normal rats. Gut 1999, 44, 394. [CrossRef]
- Cicenia, A.; Santangelo, F.; Gambardella, L.; Pallotta, L.; Iebba, V.; Scirocco, A.; Marignani, M.; Tellan, G.; Carabotti, M.; Corazziari, E.S.; et al. Protective Role of Postbiotic Mediators Secreted by Lactobacillus rhamnosus GG Versus Lipopolysaccharide-induced Damage in Human Colonic Smooth Muscle Cells. In Proceedings of the 8th Probiotics, Prebiotics & New Foods for Microbiota and Human Health meeting, Rome, Italy, 13–15 September 2015; pp. S140–S144. [CrossRef]
- Nakamura, F.; Ishida, Y.; Sawada, D.; Ashida, N.; Sugawara, T.; Sakai, M.; Goto, T.; Kawada, T.; Fujiwara, S. Fragmented Lactic Acid Bacterial Cells Activate Peroxisome Proliferator-Activated Receptors and Ameliorate Dyslipidemia in Obese Mice. J. Agric. Food Chem. 2016, 64, 2549–2559. [CrossRef]
- Chambers, E.S.; Viardot, A.; Psichas, A.; Morrison, D.J.; Murphy, K.G.; Zac-Varghese, S.E.; MacDougall, K.; Preston, T.; Tedford, C.; Finlayson, G.S.; et al. Effects of targeted delivery of propionate to the human colon on appetite regulation, body weight maintenance and adiposity in overweight adults. Gut 2015, 64, 1744–1754. [CrossRef]
- Irving, A.T.; Mimuro, H.; Kufer, T.A.; Lo, C.; Wheeler, R.; Turner, L.J.; Thomas, B.J.; Malosse, C.; Gantier, M.P.; Casillas, L.N.; et al. The immune receptor NOD1 and kinase RIP2 interact with bacterial peptidoglycan on early endosomes to promote autophagy and inflammatory signaling. Cell Host Microbe 2014, 15, 623–635. [CrossRef]
- Dinic, M.; Lukic, J.; Djokic, J.; Milenkovic, M.; Strahinic, I.; Golic, N.; Begovic, J. Lactobacillus fermentum Postbiotic-induced Autophagy as Potential Approach for Treatment of Acetaminophen Hepatotoxicity. Front. Microbiol. 2017, 8, 594. [CrossRef]
- Varian, B.J.; Poutahidis, T.; DiBenedictis, B.T.; Levkovich, T.; Ibrahim, Y.; Didyk, E.; Shikhman, L.; Cheung, H.K.; Hardas, A.; Ricciardi, C.E.; et al. Microbial lysate upregulates host oxytocin. Brain Behav. Immun. 2017, 61, 36–49. [CrossRef]
- Imperial, I.C.V.J.; Ibana, J.A. Addressing the Antibiotic Resistance Problem with Probiotics: Reducing the Risk of Its Double-Edged Sword Effect. Front. Microbiol. 2016, 7, 1983. [CrossRef]
- Newburg, D.; Ko, J.; Leone, S.; Nanthakumar, N. Human Milk Oligosaccharides and Synthetic Galactosyloligosaccharides Contain 3’-, 4-, and 6’-Galactosyllactose and Attenuate Inflammation in Human T84, NCM-460, and H4 Cells and Intestinal Tissue Ex Vivo. J. Nutr. 2015,
- Dunand, E.; Burns, P.; Binetti, A.; Bergamini, C.; Peralta, G.H.; Forzani, L.; Reinheimer, J.; Vinderola, G. Postbiotics produced at laboratory and industrial level as potential functional food ingredients with the capacity to protect mice against Salmonella infection. J. Appl. Microbiol. 2019, 127, 219–229. [CrossRef]
- Morisset, M.; Aubert-Jacquin, C.; Soulaines, P.; Moneret-Vautrin, D.A.; Dupont, C. A non-hydrolyzed, fermented milk formula reduces digestive and respiratory events in infants at high risk of allergy. Eur. J. Clin. Nutr. 2011, 65, 175–183. [CrossRef]
- Thibault, H.; Aubert-Jacquin, C.; Goulet, O. Effects of Long-term Consumption of a Fermented Infant Formula (with Bifidobacterium breve c50 and Streptococcus thermophilus 065) on Acute Diarrhea in Healthy Infants. J. Pediatric Gastroenterol. Nutr. 2004, 39, 147–152. [CrossRef]
- Perrin, V.; Fenet, B.; Praly, J.-P.; Lecroix, F.; Dung Ta, C. Identification and synthesis of a trisaccharide produced from lactose by transgalactosylation. Carbohydr. Res. 2000, 325, 202–210. [CrossRef]
- Esposito, S.; Soto-Martinez, M.E.; Feleszko, W.; Jones, M.H.; Shen, K.-L.; Schaad, U.B. Nonspecific immunomodulators for recurrent respiratory tract infections, wheezing and asthma in children: A systematic review of mechanistic and clinical evidence. Curr. Opin. Allergy Clin. Immunol. 2018, 18, 198–209. [CrossRef]
- Nylund, L.; Nermes, M.; Isolauri, E.; Salminen, S.; de Vos, W.M.; Satokari, R. Severity of atopic disease inversely correlates with intestinal microbiota diversity and butyrate-producing bacteria. Allergy 2015, 70, 241–244. [CrossRef] [PubMed]
- Bunyavanich, S.; Shen, N.; Grishin, A.; Wood, R.; Burks, W.; Dawson, P.; Jones, S.M.; Leung, D.Y.M.; Sampson, H.; Sicherer, S.; et al. Early-life gut microbiome composition and milk allergy resolution. J. Allergy Clin. Immunol. 2016, 138, 1122–1130. [CrossRef]
- Khan, I.; Ullah, N.; Zha, L.; Bai, Y.; Khan, A.; Zhao, T.; Che, T.; Zhang, C. Alteration of Gut Microbiota in Inflammatory Bowel Disease (IBD): Cause or Consequence? IBD Treatment Targeting the Gut Microbiome. Pathogens 2019, 8, 126. [CrossRef] [PubMed]
- DeWeerdt, S. How baby’s first microbes could be crucial to future health. Nature 2018, 555, S18–S19. [CrossRef] [PubMed]
- Gou, W.; Fu, Y.; Yue, L.; Chen, G.-d.; Cai, X.; Shuai, M.; Xu, F.; Yi, X.; Chen, H.; Zhu, Y.J.; et al. Gut microbiota may underlie the predisposition of healthy individuals to COVID-19. medRxiv 2020. [CrossRef]
- Scheiman, J.; Luber, J.M.; Chavkin, T.A.; MacDonald, T.; Tung, A.; Pham, L.-D.; Wibowo, M.C.; Wurth, R.C.; Punthambaker, S.; Tierney, B.T.; et al. Meta-omics analysis of elite athletes identifies a performance-enhancing microbe that functions via lactate metabolism. Nat. Med. 2019, 25, 1104–1109. [CrossRef] [PubMed]